Road Surface Erosion
In NetMap, road surface erosion is predicted using one or more of three methods: (1) READI - Road Erosion and Delivery Index, a published model that that uses road hydrologic connectivity to streams and other drain points, and that predicts optimized locations for new drains and surfacing to maximize sediment delivery reductions, (2) WEPP road model, and (3) GRAIP-Lite.
An example of how the WEPP road erosion model was applied in the Clearwater basin in western Montana is available below.
Example Application and Use:
The application of NetMap in the Clearwater River watershed of western Montana (1016 km2, 250,000 acres) represents a demonstration analysis of how the NetMap community science system can be applied to restoration planning. Funded by the South West Crown Collaborative Forest Landscale Restoration Project (CFLR) and the Clearwater Resource Council (CRC), this NetMap application utilized a 10-meter digital elevation model, a road layer provided by the Ecosystem Management ReseFieldh Institute (EMRI), information on fire risk, watershed function and anticipated habitat condition from the Lolo Forest (USFS) and distribution of cutthroat and bull trout by Montana Fish Wildlife and Parks (MTFWP) to explore the potential utility in road related restoration and conservation management.
The objective of this analysis is to evaluate the road network in the Clearwater basin in support of restoration planning to demonstrate a process of prioritization for: 1) improving road drainage and reducing surface erosion to valuable stream habitat (e.g., high quality fish habitat); 2) improving fish passage at road crossings; 3) stratifying roads for effectiveness monitoring; and 4) extrapolating and/or forecasting basin wide effects of road restoration programs.
The objective of the NetMap analysis of road surface erosion is to identify road segments that have a high likelihood of producing large amounts of fine sediment and delivering that sediment to high value streams and fish habitat. Road surface erosion can be reduced by increasing the number or frequency of cross drains (thereby reducing the effective length of overland flow on a road surface) and improving road surface materials (such as placement of gravel on a native soil surface). Reducing road surface erosion can be reduced by placement of rolling dips and other secondary drainage structures such as ‘open tops” (Fig. 1). Continuing road maintenance can be expensive and in recent years managers have seen budgets severely limit their capacity for that work. In some cases managers are abandoning, storing, or decommissioning roads in efforts to remove their long-term impacts in critically important areas. Because road restoration, including obliteration and recontouring, also can be very expensive (though it does limit long term maintenance) it is increasingly important to be able to focus limited restoration funds where they can be most useful.

Figure 1. (Top) Long stretches of road can be hydrologically connected, via overland flow. In the Clearwater River basin that can lead to considerable surface erosion and the delivery of both fine sediments and other materials (nutrients) to streams. (Bottom) The use of cross drains (a form called ‘open tops’ in the photo) can be used to decrease the length of overland flow and thus decrease the amount of surface erosion and the volume of sediment and other materials reaching stream channels. Arrows illustrate the accumulation of flow and its disruption due to the cross drain (during conditions of light rain, October 5, 2011).
Step 1: Map the distribution and values of streams and fish habitat
The MTFWP has identified the distribution of cutthroat trout and bull trout in the Clearwater basin and developed habitat ranking scores for each (Fig. 2). The GIS layers were imported into NetMap and the habitat value for cutthroat (1-10) and for bull trout (1-10) were combined to create a composite index of habitat suitability (Figure 3).
Figure 2. Habitat distribution and values for cutthroat and bull trout in the Clearwater basin (source: MTFWP; Rieman et al., 2012).
Figure 3. The stream habitat values for cutthroat and bull trout (Fig. 2) were combined into a composite score for use in NetMap.
Step 2: Analyze road drainage diversion potential.
To predict road surface erosion using WEPP requires knowledge of the hydrologic connectivity of roads or the potential length of overland flow on roads (e.g., Fig. 1). Typically, GIS road layers contain road segments that are kilometers long and are not delineated by specific physical attributes. Thus, in NetMap, GIS road layers are first broken at pixel cell boundaries thereby creating a linked population of road segments of approximately 10 m in length (when using a 10-m DEM). Next, road overland flow directions are determined for each small road segment (based on road gradient and orientation) and the small road segments are re-aggregated to create hydrologically connected road segments based on hillslope topography . In other words, road drainage (pour) points are determined based on topographic highs (ridges) and lows (swales) and further constrained by roads intersections with stream channels (in this analysis all road-stream crossings are assumed to have functioning drainage structures [bridges, culverts]). In the current version of WEPP in NetMap, secondary drainage structures are not included in the analysis, although a user can set a fixed flow accumulation distance in the tool (for example, if the user knows that a specific stretch of road has secondary road drainage structures [Fig. 1] every 400 feet, they can specify that constraint when running the tool). Road hydrologic connectivity at the scale of the entire watershed is predicted to range between 10 and 2500 m (Fig. 4).

Figure 4. Predicted road hydrologic connectivity ranged between ten and 2500 meters (average 133 m). This parameter, during large storms or following fires when secondary drainage structures may be compromised, could be viewed as an index of ‘road drainage diversion potential’. The drainage diversion index could be used to identify locations where field crews could check on drainage efficacy during or after storms or following fires.
Step 3: Analyze road surface erosion potential, point sources to streams.
The WEPP road analysis tool in NetMap can be run at any spatial scale, ranging from a single road segment (average 130 m in the present analysis) to the entire watershed encompassing 16,418 individual segments. In this demonstration analysis, WEPP was run across the entire road network in the Clearwater basin.
The following parameters were used in WEPP within NetMap: a) road hydrologic connectivity (Fig. 4); b) climate, using Seeley Lake (in the Clearwater basin) [obtained from WEPP’s stochastic climate generator, Cligen]); c) road width = 4 m; d) native rock surfacing (personal communication Shane Hendrickson, U.S.F.S., Lolo National Forest); e) high traffic (assumed constant); f) inslope, vegetated and rocked ditch; g) fill gradient and slope of 50% and 5 m; h) soil type sandy loam and i) hillslope buffer length and slope (e.g., the hillslope located between individual road segments and channels) determined for each road segment (ave. 130 m) using NetMap’s analytical capabilities. Users can change these parameter settings in subsequent runs of the model within NetMap.
Predicted annual road surface erosion ranged from near zero to 4.7 t (metric tons, 1000 kg) per year. The average predicted erosion to streams was 0.04 t/yr with a standard deviation of 0.13 t/yr (Fig. 5). The road segments (total 16,418) with the highest predicted sediment yields to streams having some combination of long road segments that are hydrologically connected (Fig.4, e.g., several hundred meters plus), steeper gradients, and close proximity to channels (limited buffers).
Figure 5. Road surface erosion (as delivered to streams) in the Clearwater basin ranged from very low (near zero) to a maximum of 4.7 tons/year. In the figure, individual road segments are color coded according to predicted annual sediment yield. Predicted sediment yields to streams are sensitive to the hillslope distance and gradient (e.g., buffer) from individual road segments to streams, as illustrated in the figure (upper right).
Because WEPP within NetMap is used in the context of remote sensing data (DEMs, road layers, modeled precipitation etc.), it is informative to compare model predictions to field measurements of road surface erosion. A recent study of road surface erosion in western Montana (geology: Belt Supergroup and glacial till materials) by Sugden and Woods (2007) is used to evaluate the WEPP erosion prediction in NetMap. Study sites targeted roads of 6-7% slope and segments were freshly graded. Plot measured road surface erosion (hillslope buffer is not considered and hence total sediment is not limited by partial delivery to streams) ranged from 2.1 to 9.9 t/ha/yr (average 5.4 t/ha/yr), to a maximum plot value of 97 t/ha/y r (Sugden and Woods 1997).
Using an average road segment length in NetMap of 133 m and an average road width of 4 m (0.05 ha) produces a WEPP average sediment yield from roads of 0.8 t/ha/yr with a maximum of 67 t/ha/yr. Since WEPP predicts sediment delivered to stream channels, the results between the two approaches are not directly comparable. For instance, the WEPP predictions are less (average factor of 6) than the measured erosion rates. The measured results do not consider delivery to streams and thus do not account for the length or slope of a vegetated buffer that would tend to limit sediment delivery to streams. In WEPP, the inclusion of an approximate 100 m vegetated buffer (between the road and the stream) can reduce predicted erosion (delivered to the stream) by a factor of eight (Elliot et al. 1999).
Other factors that could contribute to disparities between predicted and observed road erosion include the climate (WEPP that used Seeley Lake RS average annual precipitation of 535 mm/yr compared to field measured winter (May-October) precipitation of about 180 mm/yr [2002-2004] in the study of Sugden and Woods [2007]), traffic levels and time since grading. Nevertheless, considering the sediment reduction effect of buffering (that acts to reduce WEPP predictions by at least several fold), the results between the predicted erosion to streams (WEPP) and that measured in the plot studies (both as a mean and maximum) are reasonably close (Table 1), particularly in the context of erosion modeling accuracy (Reid and Dunne 1996); moreover, the median values between the two studies are quite similar [NetMap-WEPP 0.18 t/ha/yr compared to Sugden and Woods 0.16 t/ha/yr]). This indicates that WEPP, as applied within NetMap in the Clearwater basin, provides a practical screening tool to identify road segments that are potentially important contributors of eroded sediment (and other materials) to streams (Fig. 5).
In NetMap, there are no zero value WEPP road surface erosion predictions, although many values are very low and close to zero. It is likely that many road segments will have road surface erosion values of zero, because many segments are hydrologically disconnected from streams including having wide buffers (hundreds of meters). Based on field knowledge, predicted road surface erosion could be manually truncated at zero based on certain physical conditions in a GIS.
Table 1. Comparison between WEPP prediction of surface erosion and field measurements (Sugden and Woods 2007).
Source
|
Average
|
Median
|
Maximum
|
WEPP in NetMap
(Clearwater basin)
|
0.8 t/ha/yr1
|
0.18 t/ha/yr
|
67 t/ha/yr
|
Sugden and Woods (2007)
(western Montana)
|
5.4 t/ha/yr2
|
0.16 t/ha/yr
|
96.7 t/ha/yr
|
1 Includes the effect of buffering from road segments to nearest stream channel that can reduce the amount of predicted erosion (sediment delivery) by a factor of 8 in 100 m buffer width.
2 Does not include the effect of buffering, based on plot studies only.
There can be several sources of error in NetMap’s application of WEPP. One of the most significant is the misalignment of roads in the GIS. Road lines in GIS are typically digitized from aerial photography or from other map products. An error in road placement in a GIS and on a corresponding digital elevation model can lead to spuriously high road gradients, since road gradients in NetMap are derived from elevations of the grid cells located at each end of a road segment (road gradient = the difference in elevation/road segment length) (Fig. 6). In the Clearwater, approximately 10% of the road segments have slope gradients in excess of 12%, a likely upper limit for roads with mixed duty in timber harvest and recreation. Exaggerated road gradients can lead to exaggerated road surface erosion predictions in WEPP.
In NetMap’s WEPP tool, a user can specify a maximum road gradient to reduce some of the road gradient error due to misalignment problems. Ideally, however, it will be important to verify the correct alignment of roads in a GIS and correspondingly on the DEM so that the most accurate predictions of road surface erosion can be produced in NetMap. In addition, road layers should be updated to reflect the most current status of the road use (active, decommissioned, storage).
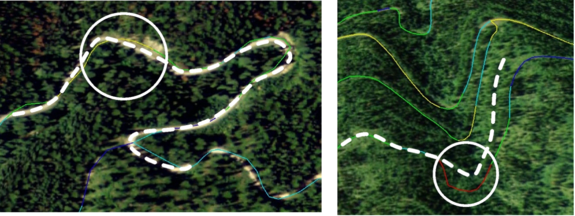
Figure 6. Colored lines represent GIS roads draped onto a Google Earth image in the northeastern corner of the Clearwater basin. Dashed white line overlays upon exact road locations on the satellite imagery. Road misalignments are highlighted by the circles. Exaggerated road gradients (considerably in excess of 12%) can lead to exaggerated road surface erosion predictions using WEPP. Another issue is that some of the roads contained within the GIS layer used in this analysis are already decommissioned but are not indicated as such in the GIS road layer.
It is feasible to use the results of the road erosion predictions in isolation to identify potential hotspots of road surface erosion to streams. Using Fig. 5, one could seFieldh for the road segments with the highest predicted road erosion given the caveat about road alignment issues in the GIS (Fig. 6). An analyst could create a table in ESRI FieldMap (in the NetMap watershed database) that ranks road segments by the predicted erosion contribution. Such as list could be used to prioritize road restoration activities (including increasing road drainage structures [Fig. 1], road reconstruction efforts [move road away from streams and floodplains – see later in the report], road abandonment or decommissioning and road storage). The field component of this analysis (recommended) would need to verify road parameters (that were used in the WEPP predictions) in addition to observations or measurements of road surface erosion and sediment delivery to streams to validate the predictions.
Step 4: Examine overlaps between road surface erosion potential and fish habitat.
Given the spatial variability of habitat value and sensitivity in the Clearwater basin (Fig. 3), it could be effective to couple road erosion predictions with indices of habitat condition. This is done by using the automated tool in NetMap that seFieldhes for spatial overlaps between user specified thresholds for erosion magnitude and habitat quality.
Using the WEPP interface in NetMap, an analyst aggregates predicted road surface erosion into stream channels (individual road segment detects which individual channel reach it drains into, either through a vegetated buffer or because the road directly intersects a stream). In some cases more than one road segment will drain into any individual stream segment. The results are shown in Fig. 7.
Figure 7. In NetMap, predicted road surface erosion (Fig. 5) is routed into individual stream segments.
In this illustrative example, three thresholds of road surface erosion (in streams, e.g., Fig. 7) are used: the mean (0.11 t/yr) and the mean plus one and two standard deviations (0.38 and 0.65 t/yr). The threshold of habitat value (combined cutthroat and bull trout) was chosen to have a value of 10. A user can select whatever values they wish in NetMap. The program seFieldhes across the Clearwater watershed for matches. The number of overlaps between high surface erosion potential and high habitat potential using the three erosion thresholds yielded 392, 117 and 43 locations in the channel network that met the criteria (Table 2).
Table 2. Using NetMap’s “overlap” tool with thresholds for road surface erosion combined with a habitat score of 10 yielded spatial matches ranging in number between 392 to 43, representing 2% to 0.2% of the total stream network of 19,889 individual stream segments; see Fig. 8. Using these (illustrative and arbitrary) thresholds would identify a similar number of particularly problematic road segments that might focus prioritization of road restoration (or refinement of information)
Road surface erosion (t/yr)
|
Combined habitat score
(Figure 3)
|
Number of matches
|
>= ave. (0.11)
|
>=10
|
392 (2%)
|
>= ave. + 1SD (0.38)
|
>=10
|
117 (0.6%)
|
>= ave. + 2SD (0.65)
|
>=10
|
43 (0.22%)
|
The identified channel segments that contain both high surface erosion potential and high habitat quality (using the thresholds in Table 1) are identified within NetMap (Fig. 8).
An alternative approach is to display the map of predicted road surface erosion potential using categories of sediment thresholds (the three in Table 1) and then identify road segments (with high erosion potential) that are in close proximity to or are in spatial relationship with high quality or sensitive habitat (Fig. 9).
Figure 8. NetMap’s overlap tools are used to identify stream segments where high road surface erosion potential (>=ave. + 1 SD, Fig. 5) overlaps with high quality habitat (>=combined score 10).
Figure 9. An analyst creates maps of road surface erosion using specific thresholds and then visually relates those to specific fish habitat segments. In this approach multiple road segments can be isolated for treatment or further field based refinement and validation.
Step 5: Analyze cumulative road surface erosion potential.
The preceding analysis targeted individual road segments as the appropriate scale for road restoration. However, users may wish to consider the effects of entire road networks (but circumscribed by specific sub watershed boundaries) with respect to erosion and impacts to fish habitat or other issues such as downstream nutrient loading linked to erosion. In this approach, NetMap aggregates the stream segment scale road erosion (Fig. 7) downstream revealing spatial patterns of road erosion at any spatial scale in a watershed as defined by the stream network (Fig. 10).
The map of cumulative road erosion potential in streams can be viewed in the context of the road segments that produce the cumulative sediment supply, and thus entire road networks within individual subbasins can be considered in terms of a road restoration program (Fig. 10).
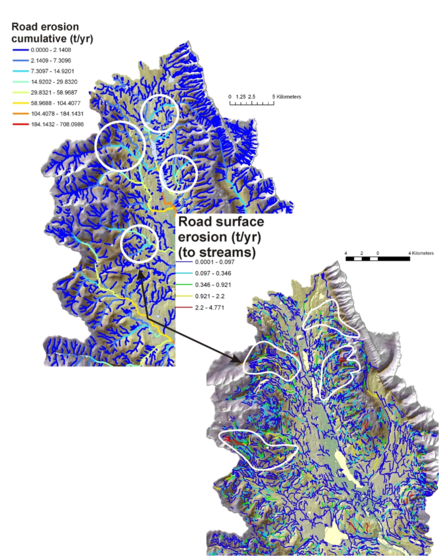
Figure 10. (Upper) Road surface erosion (delivered to streams) is aggregated (routed) downstream revealing patterns of cumulative road erosion at any spatial scale in a watershed defined by the stream network. Analyses such as this could help clarify and test questions about cumulative downstream loading associated with nutrients or other materials that could be linked to road erosion. (Lower) Cumulative patterns of road erosion (in streams) can be considered in the context of the road segments that create those patterns. The white lines (Lower) circumscribe entire road networks within individual contributing subbasins that are responsible for cumulative road surface erosion, particularly for cumulatively high values. Within individual subbasins, a restoration program could target groups of road segments that have the predicted highest road erosion potential.