Floodplain/Terrace/Alluvial Fan Mapping
Note: NetMap’s updated (Summer, 2019) floodplain/valley floor mapping tool uses an improved algorithm that more accurately delineates variations in floodplain and valley floor morphologies, including identifying potential off-channel fish habitats (that may currently be disconnected), and other low-lying areas, such as potential wetlands.
The output polygons are provided by TerrainWorks in new (post spring, 2019) virtual watershed datasets and they also exist for older LiDAR and 10m-based NetMap datasets (contact us). You may also apply the new mapping tool with older datasets to create your own floodplain/valley floor map polygons, although adequate computer power is required, and it could take more than a day (e.g., run over the weekend). See Floodplain Tool 2.0.
Floodplain Tool 1.0 - Parameter Description: The extent of the floodplain is identified as both a polygon and a Field Attribute in the stream layer in NetMap. The spatial extent of the floodplain is determined by an elevation above the channel (defined by the user) in units of bankfull depths or by an absolute elevation, and by the search radius (of valley elevations) adjacent to the channel. For additional information see also: http://www.hydrol-earth-syst-sci.net/15/2995/2011/ .
Data Type: Line (stream layer) and polygon
Field Name: Line attribute = FP_WIDTH; Polygon = Flood_, _ is the user specified number of bankfull depths form which to map floodplains; Common Name: Floodplain Width
Units: meters
NetMap Module/Tool: Fluvial Processes/Fluvial Processes/Floodplain/Terrace/Alluvial Fan Mapping
Note: Mapping floodplains using polygons provides valuable information about near channel environments. However, more detail mapping of floodplains and other valley floor features is available using NetMap's other floodplain mapping tool, shown here. Use of gridded data, particularly using LiDAR, provides greater spatial resolution.
Model Description:
To characterize valley-floor surfaces in NetMap, DEM cells are classified according to elevation above the channel. Each cell within a specified search radius of a channel (a multiplier of bankfull widths) is associated to the closest channel cell, with distance to the channel weighted by intervening relief. Valley-floor DEM cells are associated with specific channel segments that are closest in Euclidean distance and have the fewest and smallest intervening high points. The elevation difference between each valley floor cell and the associated channel location is normalized by bankfull depth or by the absolute elevation above the channel. This procedure is repeated for every channel segment. For additional information on the use of NetMap’s floodplain mapping tool, see: http://www.hydrol-earth-syst-sci.net/15/2995/2011/ .
The floodplain tool in NetMap allows the user to specify the height about the channel from which to estimate floodplain (or valley) width in units of bankfull depths or to using a fixed elevation above the channel (Figure 1). Floodplain or valley width can vary from the right to left sides of the channel. First, the user selects the height above the channel from which to measure floodplain or valley width, either in terms of drainage area-scaled bankfull depths or in terms of a fixed elevation (Figure 2). Since the DEM typically does not account for the bottom of a stream channel, a bankfull depth of one could be used to define floodplains, although the Rosgen stream classification system suggests a bankfull depth of two. It is recommended that a user make field observations (and use aerial photographs) to determine an appropriate height above the channel to measure floodplain width (and an appropriate search radius, see below). The tool calculates floodplain width as a reach attribute and as a floodplain polygon. The polygon visually indicates that differences in floodplain width from the right to left sides of the channel.
Other tool options include selecting only one channel for the analysis (a channel in NetMap is defined as a linked set of stream segments that begin at the upper most channel segment [of any particular tributary] and that extends downstream until they intersect a channel [at a confluence] that has a larger drainage area). This option is designed to help clarify the floodplain extent along a single channel without the complicating and overlapping (floodplain) influences of intersecting tributaries (e.g., floodplains from mainstem channels and tributaries can interdigitate and overlap); this described in more detail below.
Figure 1. A sketch showing the location of the active floodplain within a valley that contains a small stream at summer low flow. A user selects an elevation above the channel from which to map floodplain width in units of bankfull depths or by using an absolute elevation above the channel (same for all segments regardless of channel size).
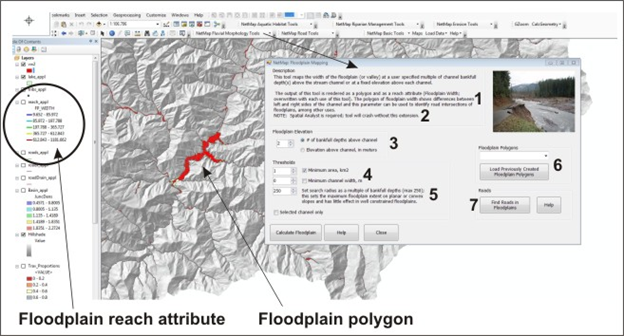
Figure 2. NetMap’s floodplain mapping tool: (1) The tool creates both an reach segment attribute (floodplain width) that can be used with a host of other tools such as predicting aquatic habitats, identifying roads in floodplain, and mapping channel confinement; the tool requires Spatial Analyst (2), although later versions of the tool (later 2013) will not require it. (3) A user selects the number (including fractions) of bank full depths or an absolute elevation above the channel from which to calculate floodplain width. (4) User defined thresholds include a minimum basin area (km2) to include in the mapping exercise or a minimum channel width, in meters. (5) Setting the search radius (in multiples of bankfull widths) allows a user to limit the floodplain extent on planar or convex landforms, such as on alluvial fans. This option has little effect on moderate to well constrained floodplains (see Figures below). (6) Floodplain polygons are saved and can be reloaded; however, everytime the tool is run it overwrites the floodplain width attribute in the attribute table. (8) Roads in floodplains can be quickly identified; this requires a road layer (Figure 5). Locating road segments in floodplains is also a tool in the Road Module.
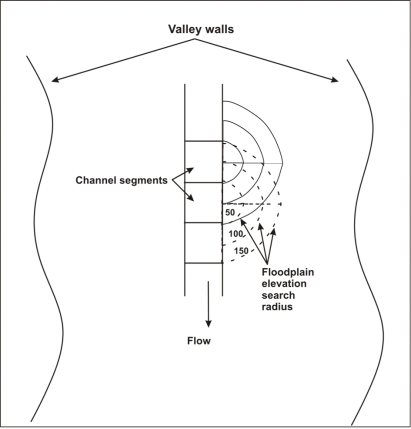
Figure 3. The floodplain tool can be adjusted to search valley floor elevations at varying distances from the channel segment, shown here at 50, 100 and 150 times bankfull width. In channels with floodplains that are relatively confined, search radius may not be a significant factor. In channels on planar surfaces that are not incised or in channels on alluvial or debris flow fans that occupy the highest elevation areas, search radius can be an important factor. See Figure 4.
An adjustable parameter is the radius over which the floodplain model searches for elevations less than the given elevation above the channel in units of bankfull depth, or an absolute elevation (Figure 4). The search radius associated with each channel node or channel segment is defined in terms of numbers of bankfull depths. Where a channel is not incised and or where the channel is located on an elevated surface relative to areas adjacent to it (convex shape), the floodplain tool can greatly overpredict the actual floodplain extent, if a large radius was used (e.g., 250 m). This could occur on planar valley floors where the channel is not incised or on alluvial or debris flow fans where the channel occupies the highest elevation area, and where the fan landform drops in elevation on either side of the channel. In moderate to well confined floodplains, varying search radius will have little to no effect. An example below illustrates how a variable search radius width leads to variable width floodplain zones. If an analyst is unsure what search radius to use (and or what elevation above channel to use) it is recommended that she run a series of scenarios to evaluate the predictions and compare them with field data or local knowledge (see also Figure 5).
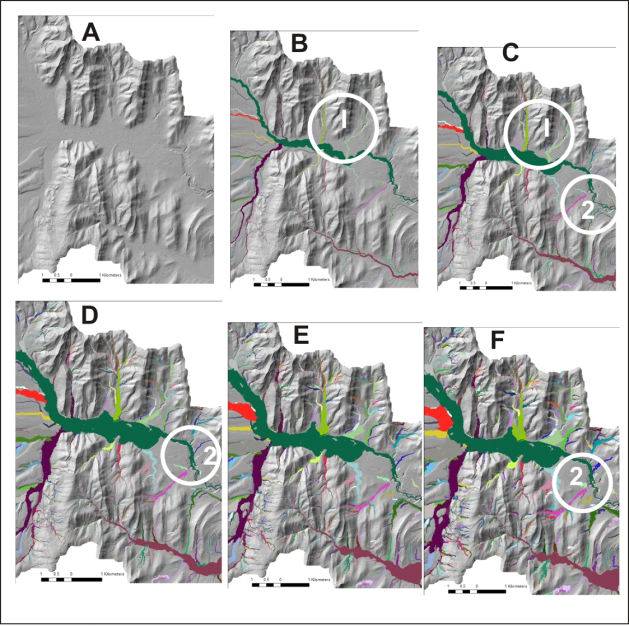
Figure 4. The floodplain search radius (in units of bankfull widths) in a watershed (A) in Alberta Canada (1m LiDAR) was varied from 10 (B), 25 (B), 50 (C), 100 (D), 200 (E) and 250 (F). Smaller radius (10 or 25 ) is more appropriate for predicting floodplains on alluvial fans and planar (non incised) valley floors (1) and in relatively confined valleys with narrow floodplains (2). See Figure 5 about how varying floodplain search radius can be used to identify landforms.
Figure 5. Varying the search radius (Figure 3) can be used to tentatively identify various landforms as shown here in Alberta Canada (using 1 m LiDAR).
Because tributary floodplains can overlap and interdigitate with mainstem floodplains, it can be useful to apply the floodplain mapping tool to individual channels. A “channel” in NetMap is defined as a linked set of stream segments that begin at the upper most channel segment (of any particular tributary) and that extends downstream until they intersect a channel (at a confluence) that has a larger drainage area. The mapped floodplains in a single selected channel are much easier to interpret than the mix of tributary and mainstem floodplains at the scale of entire networks (Figure 6).
Certain patterns can emerge when an analyst applies the floodplain tool using a range of search radii. Depending on the landscape in question, smaller radius search areas may be more applicable to small channels located on planar valley floors (non incised) or on alluvial fans. In these areas, increasing the radius of the search area will result in larger (wider) floodplains being predicted (Figure 8). In other areas, increasing the search radius will have little to no effect, such as in confined valleys with small floodplains. Thus, varying the search radius can be used to identify certain types of landforms (Figure 9).
Figure 6. (A) Mapped floodplains for both mainstem and tributaries. Note how tributary and mainstem floodplains can overlap. (B) Mapped tributaries for the mainstem channel only (Hoh River, Olympic Peninsula, WA). Note how delineated floodplains are "slices" associated with individual NetMap 100m channel reaches.
Figure 7. Floodplain at one multiple of bankfull depth (1x) generally identifies the active channel. Floodplain at two multiple of bankfull depth (2x) generally maps the lowest, most active floodplain, commonly thought to be inundated by the two-year flood (Dunne and Leopold 1978, Rosgen 1996). 3x floodplain is likely inundated by low frequency, high magnitude floods. 4x floodplain may represent an alluvial terrace or toeslopes of hillsides. Ditto for 5x and beyond.
Figure 8. NetMap's floodplain mapping tool, particularly using LiDAR, can be used to map the elevation diversity of floodplain surfaces (see floodplain mapping tools).
Figure 9. Although mapping floodplains using 10m DEMs (NetMap virtual watershed) is adequate (left panel), LiDAR (1-2m) provides considerably more detail and variability in floodplains.
Figure 10. An example of how NetMap’s floodplain mapping tool can be used to examine variable floodplain surfaces using a range of bankfull depths above the channel (e.g., 1 x bankfull depth, 2x, and 3x etc.). Shown above is the present day (diked and regulated) channel in a basin in Northern Spain with NetMap’s predicted floodplains shown at 1x, 2x, and 3x bankfull depths. NetMap was used to develop a catchment scale perspective for river restoration in Spain (http://www.hydrol-earth-syst-sci.net/15/2995/2011/ ).
Figure11. NetMap's floodplain mapping tool, particularly using high resolution LiDAR DEMs, can be used to identify the current floodplain and historical floodplains, now abandoned due to diking, stream cleaning, agriculture and channel incision; analysis in this figure uses 2.5 m LiDAR in the Nehalem watershed in northwestern coastal Oregon.
Figure 12. NetMap estimates floodplain width (individual left and right side or combined sides) and that channel segment data are stored in the reach attribute table in ArcGIS.
Figure 13. NetMap contains a tool for quickly identifying where roads may intersect floodplains. Refer to the NetMap’s Road Module. Field validation is recommended.
Technical Background
Floodplains typically lie at, or somewhat above, bankfull stage (Dunne and Leopold, 1978). In practice, zones of frequent inundation are defined by an elevation above the channel equivalent to two bankfull depths (Rosgen, 1996, Castro, 1977). To illustrate a wide range of flow inundation-valley topography relations in NetMap, multiple valley widths can be calculated, e.g., 2x, 3x, and 5x bankfull depths (Figure 9).
Geologic controls and major slope movements can create variations in floodplain (and valley width) and shape. Constrained (narrow) valleys typically lack sediment storage while unconstrained (wide) valley segments act as storage reservoirs of alluvial sediment, often creating wide, more complex floodplains within the fluvial landscape (Grant and Swanson, 1995, McDowell, 2001). At the upstream transition from unconstrained to constrained valley segments, a bottleneck in the transfer of sediment can occur, often enhanced by logjams. Increased sediment storage at the transition promotes heightened connectivity between channels and adjacent valley floors. The transition from unconstrained to constrained segments also enhances hyporheic downwelling (Figure 11). At the downstream transition from constrained to unconstrained valley segments, increased sediment deposition, flow divergence, and hyporheic upwelling can occur (Edwards, 1998). Thus, there is typically greater channel-valley connectivity and hence larger fluvial landscapes immediately above and below valley constrictions, with increased occurrence of floodplains, side channels, and riverine wetlands (Baxter, 2001). Alternating constrained and unconstrained river segments promote patchy heterogeneity in fluvial processes and riparian environments, a pattern often referred to as a “string of pearls” (Standford and Ward, 1988) (Figure 12).
Constrained channel segments are often characterized by rapids and bedrock influenced morphology resulting in channels being less sensitive to changes in discharge or fluctuating sediment supply. In contrast, unconstrained floodplain segments store greater volumes of sediment and wood, contain a greater diversity of low-gradient habitats, and are more sensitive to disturbances. Typically, wide valley floors promote formation of wider channels and floodplains (Grant and Swanson 1995, Benda et al. 2003b), higher sinuosity (McDowell 2001), deeper pools (McDowell 2001), greater side channels (Baxter 2001), increased gravel substrate (Perkins 2000), and valley-paralleling side channels.
River floodplains are one of the most geomorphically and ecologically significant components of the fluvial system. The active floodplain is a transition landform between a stream or river and its valley. Floodplain landforms are often interdigitated with alluvial fans and higher elevation modern and ancient terraces (Figure 11).
Locations of floodplains and their association with tributary confluences (see ‘Confluence Environments’ in NetMap’s Fluvial Morphology Module’) can be used to identify provisional biological hotspots (Figure 13). To view how NetMap’s floodplain and tributary confluence tools can be used in reconstructing fluvial landscapes for planning restoration, see: http://www.hydrol-earth-syst-sci.net/15/2995/2011/
Polygon maps of floodplains are also used in NetMap to identify roads that intersect floodplains (Figure 5).
Figure 11. An example of variable floodplain widths and the diminution of floodplains in upstream parts of a mountain stream network (From Swanson, F.J., and James, M.E., 1975, Geology and geomorphology of the H.J. Andrews Experimental Forest, Western Cascades, Oregon: Pacific Northwest Experiment Station, USDA Forest Service ReseFieldh Paper PNW-188, 14 p.)
Figure 12. The alternating pattern of constrained valleys (canyons) and unconstrained segments (floodplains) are important in controlling the types, abundance, and spatial distribution of aquatic and riparian habitats within watersheds. Unconstrained floodplain segments generally offer higher quality and more diverse habitats compared to canyons. Discontinuous pattern of floodplain segments in rivers have been referred to as a “string of pearls” (Ward et al. 2002). Figure adapted from Ward et al. 2002.
Figure 13. NetMap is used to predict floodplain widths across entire drainage basins and hence the amounts and locations of canyon and floodplain segments. These maps, in addition to predictions of geomorphically significant confluences, are used to identify areas of the potentially best habitats (biological hotspots). Because of the resolution of 10-m digital elevation data, the relative variation in floodplain and valley widths is more accurate than the absolute measures.